Over the rainbow Why understanding full value-chain carbon intensity is trumping the colour of hydrogen
February 2024
Authors
Labelling hydrogen by colour is a popular way of differentiating its production process. The hydrogen ‘rainbow’ includes brown hydrogen, made using coal, and grey hydrogen, produced from natural gas. Blue hydrogen is grey or brown hydrogen produced using carbon capture and storage (CCS) to cut carbon dioxide emissions, while green hydrogen, produced from water through electrolysis fuelled by renewable power, offers the potential for near zero emissions.
As momentum builds around low-carbon hydrogen, the industry is having to look past colour labels. The future of low-carbon hydrogen hinges on governments putting in place regulations, subsidies and other incentives that are increasingly tied to the carbon intensity ‒ rather than the colour ‒ of the hydrogen produced.
Calculating hydrogen’s carbon intensity is complex. For green (electrolytic) hydrogen, emissions can range from almost zero to levels beyond those of brown hydrogen. Green hydrogen is, in principle, made using 100% renewable energy. In practice, however, what is described as ‘green’ can also be produced using power from a grid that relies heavily on fossil fuels.
What’s more, hydrogen's carbon intensity isn’t limited to its production. With over 40% of announced project capacity targeting exports, it is important to understand its full life-cycle emissions, including processing and transportation. The European Union (EU) is already using full-cycle emissions to assess eligibility for its incentives and regulatory compliance, and other hydrogen markets are likely to follow suit. But different importers may have very different incentives and standards, leading to a two-tiered low-carbon hydrogen market.
The industry, therefore, requires ever more accurate project-level certification of carbon intensity as the market for low-carbon hydrogen evolves. With the sector requiring massive levels of capital investment and subsidies to support growth in supply and demand, it is time to go beyond the rainbow and establish hydrogen’s true colours.
Deciphering the carbon intensity of the hydrogen rainbow
The global hydrogen market today is around 90 million tonnes per annum (Mtpa), almost all of it carbon-intensive grey or brown hydrogen. The volume and make up of supply is about to change dramatically. In our base-case forecast, we project production to triple to 270 Mtpa by 2050, with low-carbon green and blue hydrogen accounting for 200 Mtpa of this. In a world on course for net zero emissions by 2050, that growth would have to be even faster: our Net Zero 2050 scenario (detailed in our energy transition outlook) requires 500 Mtpa of low-carbon hydrogen by 2050.
The push for better measurement of efforts to cut emissions globally is shining a spotlight on the precise carbon intensity of different sources of hydrogen supply. Because of its potential to deliver almost carbon-free hydrogen, green hydrogen is generating the most industry interest, but it is important to look more closely at the full value chains of blue and green hydrogen.
Figure 1: Carbon intensity of the hydrogen rainbow
Assumptions: Values are for 2023. The blue low end (0.5 kgCO2e/kgH2) represents the average blue asset in Norway with 95% CO2 capture. The high end represents the average blue asset in North Dakota (USA) with 60% CO2 capture. Blue includes methane fugitive emissions, which vary by asset. For green, the low end assumes an electrolyser powered 100% by renewables and the high end assumes a grid-connected electrolyser in India.
In the case of blue hydrogen, emissions can come from upstream natural gas production, transportation, reforming and energy use. The bulk of the carbon dioxide emissions are produced in the reformer, which splits hydrogen out of hydrocarbons. In principle, almost all these emissions can be captured and stored. However, capturing more than 60% of the carbon dioxide from hydrogen production is costly and has yet to be proven at scale.
New autothermal reforming (ATR) technology can achieve 95% carbon dioxide capture at a lower cost. Unfortunately, the total emissions of ATR with 95% carbon dioxide capture could still be higher than for a steam methane reformer (SMR) with 95% capture, as it requires an energy-intensive air separation unit. Developers will have to evaluate the cost to emissions reduction potential of all emissions abatement options. Some developers will use renewable power to reduce the emissions from the electricity used in reforming and capture, but this must also be balanced against potentially higher costs.
How green is green hydrogen?
For green hydrogen, nearly all emissions are attributable to the electricity used by the electrolyser. In principle, hydrogen should be called green only if it uses 100% renewable power. However, because of the variability of renewables such as wind and solar, many electrolytic hydrogen projects around the world are planning grid connection to maximise the utilisation of electrolysers and lower hydrogen unit costs. In Wood Mackenzie’s Lens Hydrogen project tracker, at least 30% of the 565 GWe of announced or operational green hydrogen projects plan to be grid connected.
While projects able to secure all of their power supply from certifiable renewable sources will have negligible production emissions, this will not be the case for projects that require access to grid power. At the opposite end of the CI spectrum, we estimate that emissions from electrolytic hydrogen produced from 100% grid power today could be as high as 50 kgCO2e/kgH2 – worse than brown hydrogen in our study – if the electrolyser is connected to a grid dominated by fossil fuels. As grids decarbonise, carbon intensity levels will fall accordingly, reinforcing the importance of regular certification of emissions from hydrogen production.
It is also worth noting that electrolyser demand for clean power could also inadvertently lead to additional fossil-based generation to meet other demand on the grid, increasing overall emissions, especially in markets that lack rules on additionality (adding new renewable capacity alongside hydrogen production) and temporal correlation (matching renewable generation to hydrogen production).
Could a two-tier low-carbon hydrogen market emerge?
Policymakers in many parts of the world are keen to avoid a two-tier low-carbon hydrogen market and have put in place a variety of different rules on additionality, temporal correlation and the geographical location of renewables. Regulation varies significantly by country, however, and this variance risks the emergence of a two-tier market for electrolytic hydrogen.
The EU has led the way, establishing the first set of rules for electricity used to produce electrolytic hydrogen, which allow grid-connected electrolysers only under very specific conditions. The US has similarly announced stringent rules for the use of grid power and renewables in electrolysers to govern eligibility for tax credits based on carbon intensity.
Figure 2: Blue and green hydrogen emissions, 2023
Assumptions:
1) Blue hydrogen: Assuming a retrofitted SMR unit with 60% capture. Reforming emissions will vary by technology (SMR vs ATR) and whether the asset is retrofit or newbuild. For upstream, we assume the average emissions for all gas-producing assets in each country, including methane fugitive emissions. Upstream emissions and methane fugitive emissions vary by asset. Electricity for reforming and CCS is sourced from the grid, assuming an average grid intensity in each country. The grid intensity will vary by region in larger markets such as Australia and the US and will decrease over time.
2) Green hydrogen: assuming the electrolyser is powered by on–site renewables and 20% grid electricity, taking the average grid intensity. Electricity consumption assumed is 55kWh/kgH2 for the electrolyser system. The electricity consumption will vary by electrolyser technology and can range from 40 kWh/kgH2 to 60 kWh/kgH2 for an electrolyser system. Electrolyser efficiency is expected to improve over time.
Source: Wood Mackenzie Lens Hydrogen and Ammonia Service
Other major markets, such as Japan, South Korea, Canada and India, currently have less stringent rules on grid-connected electrolysers, but do require developers to have a green power purchase agreement (PPA) in place. However, the availability and deliverability of a truly green PPA remains challenging, even in the most willing markets.
In some developing economies, such as India, the rapid roll-out of renewables is struggling to keep pace with power demand growth, limiting green PPA availability for electrolytic hydrogen. In addition, markets with grid congestion face hurdles in delivering green power, despite developers having signed a PPA. In these markets, permitting some grid supply can be seen as the pragmatic approach to kickstarting the hydrogen economy.
China will inevitably play a role
Inevitably, China will also impact the outlook for electrolytic hydrogen production. The country already has 0.3 Mtpa of grid-connected electrolysers in operation, largely based on Chinese alkaline technology. Chinese alkaline electrolysers have lower limits of 20% to 50% to operate safely, meaning they require some continual electrical load. PEM technology, more commercialised by western OEMs, can operate at lower limits closer to 0%, allowing developers to mirror hydrogen production to renewable generation. But this comes at a higher cost. China’s role will be critical, with the country accounting for 57% of the current 45 GW of global electrolyser manufacturing capacity and an additional 15 GW planned in 2024.
With China’s highly competitive electrolyser OEMs seeking to dominate the global market in a similar way to its renewables and battery manufacturers, China’s low-cost and efficient alkaline electrolysers could proliferate. This could have consequences for both for technology choices and emissions. A significant expansion of grid-powered hydrogen projects operating on China’s alkaline technology across price-sensitive emerging economies could result in a two-tiered hydrogen market.
Emissions from hydrogen transport and processing
If hydrogen is produced close to the final consumer, then focusing on production emissions does a fair job of accounting for most of the emissions in the hydrogen value chain. But any future trade in hydrogen between Australia and Northeast Asia or the Middle East and Europe means significant shipping distances. And once transport is required, production emissions only tell part of the story, as unaccounted, often substantial, emissions occur through the rest of the value chain.
Many countries have already established carbon-intensity thresholds for low-carbon hydrogen. But most, including future importers such as Japan and South Korea, only count production or well-to-gate emissions. For future developers and buyers of blue and green hydrogen, it is critical to consider emissions abatement strategies across each step of the value chain.
At present, only the EU counts full-life-cycle emissions from converting, compressing, transporting and reconverting hydrogen. This creates additional challenges for hydrogen project developers seeking to export hydrogen to the bloc. Developers must manage emissions from ammonia synthesis and transportation to ensure they do not breach the EU’s threshold, while also being subject to Carbon Border Adjustment Mechanism (CBAM) rules.
Figure 3: Hydrogen carbon intensity thresholds and emissions scope
Note: Hydrogen and derivatives (ammonia, methanol and synthetic fuels) can be used in end-use sectors. Using a hydrogen derivative directly can have both cost and emissions savings.
Source: Wood Mackenzie
Because of its low density, transporting hydrogen requires compression or liquefaction – both energy- and emissions-intensive processes – or conversion into derivatives. For long distances by sea, only carriers such as ammonia and methanol offer the technological readiness to transport hydrogen at scale this decade.
Ammonia emissions
Most developers of hydrogen export projects aim to use ammonia as the carrier. Hydrogen would be converted into ammonia, shipped to a port close to the final consumer, and then cracked back into hydrogen. Although ammonia is the most promising carrier from a cost and a technology readiness perspective, its total value-chain emissions (ammonia synthesis, transportation and cracking) are significant, and could add 1-4.5 kgCO2e/kgH2 to the CI of the final product.
Figure 4: Carbon intensity of ammonia
Source: Wood Mackenzie
Ammonia is synthesised via the energy-intensive Haber-Bosch process and transported in vessels that today run almost exclusively on bunker fuel oil. Ammonia shipping emissions will vary depending on the carrier size (25,000–65,000 m3) and distance travelled.
Some sectors, such as power, can use ammonia directly, but others will need to crack ammonia back into hydrogen. Ammonia cracking requires an energy source and, typically, a stream of uncracked ammonia is combusted to provide the necessary heat for the reaction. Alternatives exist, but either way, some energy will be needed in the process, potentially generating additional emissions.
Emissions from transport and processing can make a critical difference to whether hydrogen sources can meet regulatory requirements. Green hydrogen with 20% grid supply and blue hydrogen with 60% capture do not make the cut in the EU. But even US blue hydrogen with 95% capture converted to ammonia and shipped to the EU would have a landed emissions intensity at the very limit of the European carbon intensity threshold. Cracking the ammonia back into hydrogen in the Netherlands, for example, would tip hydrogen over the edge.
Figure 5: Emissions from ammonia imported into the Netherlands, 2023
Assumptions:
1) Blue hydrogen: assuming a retrofitted SMR unit with 60% capture. Reforming emissions will vary by technology (SMR vs ATR) and whether the asset is retrofit of newbuild. For upstream, we assume the average emissions for all gas-producing assets in each country, including methane fugitive emissions. Electricity for reforming and CCS is sourced from the grid, assuming an average grid intensity in each country.
2) Green hydrogen: assuming the electrolyser is powered by on–site renewables and 20% grid electricity, taking the average grid intensity. Electricity consumption assumed is 55 kWh/kgH2 for the electrolyser system.
3) Ammonia: assuming power is sourced from the grid, taking the average grid intensity in each market.
4) Ammonia shipping: assuming ammonia is transported in a 25,000 m3 vessel running on bunker fuel oil.
5) Ammonia cracking: assuming power is sourced from the Netherlands grid.
Source: Wood Mackenzie Lens Hydrogen and Ammonia Service
Green hydrogen made using 100% renewable power and converted into green ammonia would have an emissions intensity below the EU threshold, even if shipped from Australia. But if imported hydrogen is produced using even a small amount of grid power, it could struggle to stay below EU threshold limits.
Exporters, therefore, will need to focus on technologies for reducing the emissions from ammonia, transport and processing. Ammonia production and cracking emissions can be reduced by using renewable electricity at the facilities, while shipping emissions can be reduced by operating vessels on a low-carbon fuel, including ammonia itself.
Incentives linked to an array of emissions policies
Subsidies will be vital in order to support low-carbon hydrogen supply and demand for years to come and will make or break project economics. With carbon-intensity thresholds and associated rules forming the basis of incentive frameworks in most markets, a key issue for the industry now is how far these rules will incorporate full-cycle emissions including transport and processing.
Only the EU defines carbon intensity as including emissions across the full life cycle. In the US, guidance issued by the Treasury in December 2023 sets increasingly demanding requirements for projects to be eligible for the maximum US$3/kgH2 production tax credit available under the Inflation Reduction Act. However, under the current well-to-gate scope, US green hydrogen project developers need to source renewable electricity only for their production, not for any conversion to ammonia or another derivative.
In Asia, Japan and South Korea have signalled they will gradually expand the emissions scope to ‘landed’ to include ammonia conversion and transportation emissions, though neither has yet implemented this.
It looks inevitable that project developers will require detailed certification across the value chain to sell their delivered product into an increasing number of markets. This won’t come cheap. Several bodies have emerged that are willing to certify entire hydrogen value chains for a hefty fee. And without global agreement on carbon-intensity measurements, emissions scopes, methodology and rules, developers may require multiple certificates to access different markets.
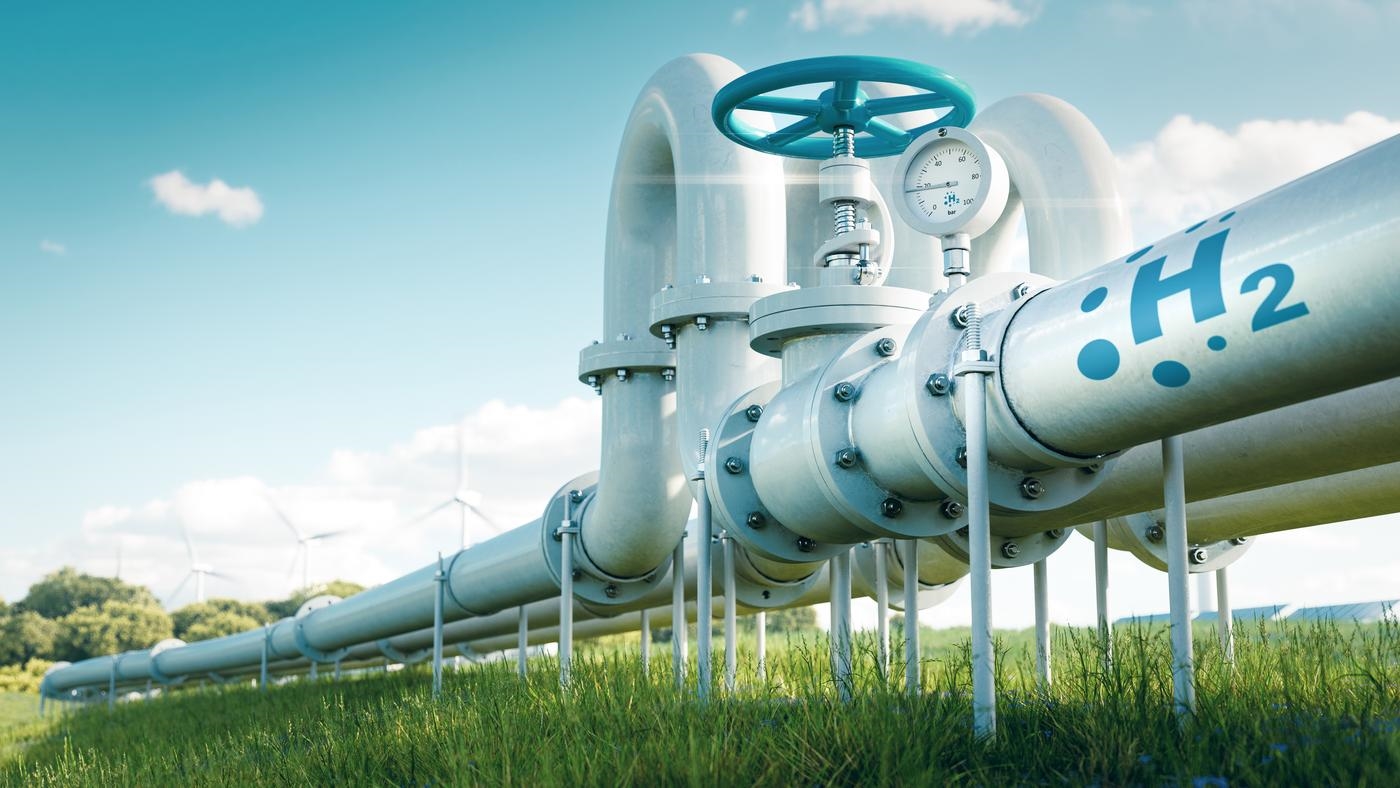
Liked this article? Join us for our third annual Hydrogen Conference
Navigate the paths to hydrogen deployment with us in London, 6-7 November.
Explore our latest thinking in Horizons
Loading...